Oesophageal injury prevention has become a major concern in the field of electrophysiology since the first case of atrio–oesophageal fistula was reported as a complication of endocardial surgical radiofrequency ablation (RFA),1 with Scanavacca et al. and Pappone et al. subsequently reporting this complication in patients who underwent percutaneous pulmonary vein isolation (PVI) for AF.2,3 It is well recognised that oesophageal wall injury, varying from erythema to ulceration, is common and has been described by endoscopy in up to 47 % of patients following PVI.4,5 The potential of atrio–oesophageal fistulas is a limiting factor for optimal ablation in a considerable number of PVI cases, as close contact between the oesophagus and the posterior left atrial wall limits the amount of energy delivered in this area.
In the vast majority of patients undergoing AF ablation, a large contact area between the left atrium (LA) and oesophagus can be observed. The mean vertical contact length is 4.4 cm and the mean distance between the anterior wall of the oesophagus and the posterior LA endocardium is only approximately 2.6 mm (range: 1.4–6.0 mm).6 In addition, the thickness of the pulmonary veins (PV) wall and PV antrum has been reported to be as little as 2–3 mm, generally thinner than the atrial myocardium at the posterior or anterior free walls (Figure 1).7 From an engineering study using a theoretical model, oesophageal injury results exclusively from thermal conduction from the atrium and is mainly influenced by the thickness of the intervening connective tissue.8 Additionally, damage of the vagal plexus coursing along the oesophagus can result in gastric hypomotility and gastroparesis.9 As such, techniques to prevent oesophageal damage during AF ablation have generated considerable interest during the last decade.
Magnitude of the Problem
Despite initial reports from intraoperative RFA that reported atrio-oesophageal fistula incidence as high as 1 %9,10 and subsequent reports from surveys reporting an incidence ranging from 0.03 to 0.5 %,11,12 the true incidence remains unknown. It is noteworthy that in larger cohorts, such as the retrospective study performed by Cappato et al., which included 45,115 catheter ablation procedures in 32,569 patients, the incidence of atrio–oesophageal fistula was 0.01 % (451 patients), with a 100 % fatality rate if left untreated.13 Strikingly, even if no oesophageal wall injury is seen (Figure 2), morphological changes of the peri-oesophageal connective tissue and the posterior wall of the LA are diagnosed in almost one-third of the patients by endosonography.14 In a more recent article published by Barbhaiya et al., results were published from a global survey regarding oesophageal perforation and fistula completed by 405 physicians who performed 191,215 AF ablations. Oesophageal perforation without fistula was reported in 0.016 % patients. Mortality in those with atrio–oesophageal fistula was 70 % and in those without fistula was 13 %, with those surviving the fistula requiring surgical procedure.15 As a result, most electrophysiologists have opted for a reduction in maximum catheter ablation power and lesion duration on the posterior LA in an attempt to avoid this devastating complication, increasing the chance of PV/posterior wall reconnection and arrhythmia recurrence.9
Risk Factors, Oesophageal Localisation and Injury Prevention
Although no major prospective studies are available delineating risk factors for the development of oesophageal damage, Martinek et al. retrospectively reported in patients diagnosed with persistent AF, who underwent additional ablation lines, had LA enlargement, were exposed to high oesophageal temperatures, received increased power during energy delivery on the posterior wall, had a short atrium-to-oesophagus distance (defined as a distance of <2 mm), were exposed to the use of nasogastric tubes, and underwent general anaesthesia to be at increased risk of oesophageal injury and stroke risk.16,17 Interestingly, Good et al. reported that the oesophagus naturally shifts sideways by ≥2 cm in most patients undergoing PVI via conscious sedation.18 These observations led to the development of intraoperative monitoring tools to prevent and/or decrease potential oesophageal damage during ablation.
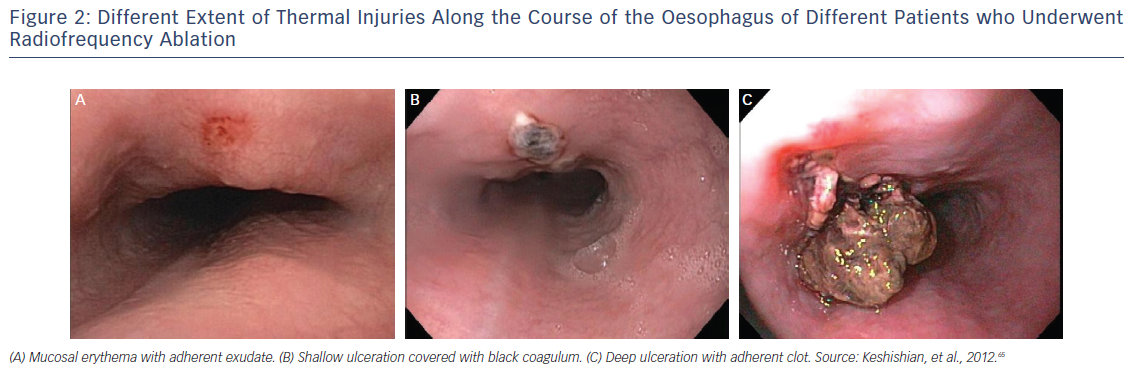
Assessing the anatomic relationship between the LA and the oesophagus can be categorised into non-real-time and real-time methods. Non-real-time methods, such as computed tomography (CT) or cardiac magnetic resonance (CMR), provide pre-procedural information about the course of the oesophagus and its location relative to the LA (Figure 1). Real-time methods provide imaging during RFA energy delivery and include repetitive fluoroscopic visualisation of the oesophageal lumen along with the help of intraluminal temperature monitoring and/or barium administration.19–21 Additionally, 2D and 3D intracardiac ultrasound,22–24 and/or anatomical delineation with a mapping/ablation catheter, are imaging modalities available to assess oesophageal position (Figure 1).25
Techniques to Prevent Oesophageal Injury
The blood supply, nerves and surface of the oesophagus can be affected when RFA is applied on the posterior wall of the LA. When performing RFA, important factors to consider are thermal conduction, increases in intraluminal temperature, magnitude and duration of local tissue heating, energy delivery, catheter tip size, contact pressure and atrial thickness.7,8,26 Different methods are available to help mitigate oesophageal injury, including intra-procedural oesophageal temperature monitoring, titration of delivered energy, mechanical displacement of the oesophagus to avoid direct damage (with the objective of increasing ablation time on the posterior wall), and using post-procedural proton pump inhibitors (PPI) and sucralfate to prevent inflammatory changes associated with these RF lesions. These will be reviewed in the following sections.
Oesophageal Temperature Monitoring
As described above, oesophageal injury appears to be mainly driven by contact force (CF), connective tissue thickness and the extent of thermal damage.8 Given the variability of energy delivery and tissue damage despite constant adjustments of the RF generator settings, several studies have examined the importance of the proximity of the oesophagus to the LA and the rise in temperature during ablation.19 Cummings et al. performed PVI in 81 patients with the use of a standard oesophageal temperature-monitoring probe, CT scan to assess oesophageal course, and endoscopic evaluation pre- and post-procedure to assess any microscopic luminal changes. As expected, oesophageal temperature was significantly higher during lesion application over the course of the oesophagus than lesions applied elsewhere (38.9 ± 1.4°C, 36.8 ± 0.5°C, p<0.01), and lesions that generated early changes of oesophageal injury had higher oesophagus temperatures than those that did not (39.3 ± 1.5°C, 38.5 ± 0.9°C, p<0.01).21 In a larger cohort of 359 patients undergoing PVI, Kuwahara et al. compared perioesophageal nerve injury leading to oesophageal dysfunction in patients receiving oesophageal temperature monitoring with a deflectable probe placed in proximity to the ablation electrode compared to those without temperature monitoring. Gastric hypomotility owing to perioesophageal nerve damage was observed in three patients in the non-monitored group, and none in the monitored group (p=0.02).27
However, oesophageal temperature monitoring is not without controversy. Deneke et al. initially suggested that routine monitoring may contribute to oesophageal damage caused by thermal effect.28 In a prospective study conducted by Muller et al., 80 patients with drug refractory or longstanding persistent AF underwent posterior wall ablation with a limited power output of less than 25 W and received either continuous oesophageal temperature monitoring or no oesophageal probe. The incidence of oesophageal injury assessed by endoscopy was higher in patients with temperature monitoring compared to those without (30 % versus 3.5 %, p<0.01) and multivariate regression analysis showed that the use of an oesophageal temperature probe is the only independent predictor for the development of oesophageal damage (OR 16.7, p<0.01).29 However, there is controversy about the existence of this so-called “antenna effect”: while Nguyen et al. reported that ablation in close proximity to metal resulted in higher temperatures in surrounding tissues leading to significant tissue heating,30 this was not confirmed in a computational modelling study by Pérez et al. in which no electrical and/or thermal interaction was found between the ablation catheter and the oesophageal temperature probe.31 According to the 2017 AF ablation consensus statement, there is general agreement that it is reasonable to use an oesophageal temperature probe during RF ablation procedures to monitor oesophageal temperature and help guide energy delivery (Class IIA, LOE-C).32 Currently, it is common practice to stop ablation when a rise of 1–2°C in intraluminal temperature from baseline occurs, or a temperature of 39–40°C is reached during ablation.
Nonetheless, the role of temperature monitoring as a sole predictor for oesophageal injury is debatable. Despite the well-established relationship between energy delivery and oesophageal temperature elevation, other factors associated with oesophageal lesion formation are at play.21,27 Tissue contact force has been described as having a key role for injury development.33 Because factors such as LA thickness and distance between the oesophagus and the LA vary among patients, they have been implicated as determinants of the amount of heat that is being transferred to the oesophagus.7,34 One of the most prevailing arguments against the use of an oesophageal temperature probe is that it can create a false sense of security. Owing to the size of the oesophagus, the ablation electrode might not align with the temperature probe, leading to a spuriously low temperature reading, which in turn could lead to increased power delivery or ablation duration on the posterior wall.35 Different probes are currently available with significant variations in temperature reading, , including multi-sensor, single-sensor and stethoscope models. Thus, the level of intraluminal temperature cut-off that can be considered safe has major variations according to the probe used (Figure 3).36 However, Tschabrunn et al. reported that multi-sensor self-expandable probes appear to provide greater sensitivity (100 % versus 60 %) and similar specificity (60 %) for the detection of oesophageal ulceration than single electrode probes.37 More evidence is needed to fully evaluate the role of oesophageal temperature monitoring in AF ablation, as well as to compare the different types of monitors and energy delivery.
Energy Delivery
Current treatment for AF requires creation of effective ablative lesions by energy delivery to a thin atrial tissue layer, with potential serious adverse events such as oesophageal damage, including ulcerations, perforation and fistulas. Factors that influence this energy delivery include impedance, power, thrombus formation, tissue CF, tissue/electrode interface temperatures and time of energy application. As stated above, thermal injury has been well described to play a key role in oesophageal damage.8 To reduce heat conduction to the oesophagus, different modalities have been tested. Martinek et al. randomised 175 AF ablation patients to one of three different techniques aimed at limiting oesophageal damage during RFA (ablation without visualisation of the oesophagus, limiting power to 25 W in the posterior wall; ablation guided by direct visualisation of the oesophagus using barium with a maximum power of 15 W; and ablation with 25 W using direct visualisation and application duration of less than 5 seconds, known as “short burns”). The authors found that oesophageal ulcerations were rare when using a “short burn” approach, defined as lesions with an output of 25 W up to a maximum of 5 seconds.16 However, despite reducing the incidence of oesophageal ulcerations, the clinical short-term success of the procedure using this approach was lower than that in the control group (51.6 % versus 58.5 %, respectively).16
It is important to note that different ablation catheters have different energy delivery efficacy, and that power, time and CF cut-offs should be individualised. For example, Deneke et al. studied the safety of a novel multipolar irrigated RF ablation catheter (nMARQ™), capable of performing “single shot” PVI through a decapolar electrode, which synchronously applies ablative energy. Despite a high effectiveness in achieving PVI, a high incidence of oesophageal lesions (33 %) was reported, especially in patients in whom a rise in intraluminal oesophageal temperature was observed.38 The incidence appeared to be higher in comparison with other ablative techniques.28,39 Given the lack of robust clinic trials for this modality and without irrefutable evidence for reduction in oesophageal injury, nMARQ AF ablation is not currently recommended in the US.38,40
Open irrigation is associated with deeper and larger lesions, as irrigated catheters minimise the risk of thrombus formation by cooling the catheter surface, thus allowing the use of higher power settings and longer durations.41 Though more efficient for tissue ablation, this may increase the risk of suffering oesophageal damage. Several attempts have been made to mitigate these untoward effects of open irrigation ablation. Sato et al. demonstrated that increasing the number of irrigation channels in an ablation catheter is associated with a slower increase in oesophageal temperature, thus providing a potential strategy to reduce the risk of oesophageal lesions.42
With non-irrigated ablation, lesion width and depth increases linearly as a function of electrode-tissue interface temperature up to 90°C. Nevertheless, coagulum formation on the catheter tip occurs with temperatures exceeding 80°C and sudden impedance rises can occur, frequently limiting the duration of RF delivery and thus the extent of energy delivery to tissue. Producing deep lesions with irrigated ablation may not be optimal in thin-walled tissues such as the posterior LA, which lies in close proximity with structures vulnerable to collateral injury, such as the oesophagus and the lungs. On the posterior LA, the deep lesions produced by irrigated ablation catheters may expose the oesophagus to much higher temperatures without increasing the success of ablation, since a sufficient endocardial lesion could be achieved with non-irrigated catheters (Figure 4).
In an experimental clinical study by Kumar et al.,43 the authors hypothesised that short duration, low-flow irrigated radiofrequency ablation of the posterior LA would allow creation of transmural lesions with a maximum diameter at the endocardial surface, without compromising safety or efficacy. Biophysical parameters and pathology measurements were compared between open irrigation at low flow (2 mL/min) versus conventional flow rates (17 mL/min) during irrigated catheter ablation in 20 swines, along with biophysical parameters in 60 patients undergoing de-novo catheter ablation for AF. Low flow ablation compared to control in swine had larger impedance decreases (median 20 Ω versus 8 Ω, p<0.001), higher incidence of loss of pace capture (44 % versus 18 %, p<0.001), more electrogram-based transmural lesions (57 % versus 9 %; p<0.001), and more visible lesions on anatomic inspection (75 % versus 41 %, p=0.007). Low flow, compared to standard higher flow irrigation, also produced a more rapid impedance drop with shorter RF applications, while achieving a higher incidence of electrical inexcitability with pacing. This was noted in both swine and human studies. Importantly, no evidence of an abrupt rise in impedance, steam pops, or coagulum formation was noted with low-flow ablation at 20–25 W and limited duration (i.e. 10–15 seconds). The greater tissue and catheter tip temperatures seen with low-flow lesions seem to be responsible for the larger impedance decreases, which may partially explain why impedance alone is not a good predictor of overall lesion size, rather a better predictor of surface heating. In the human ablation analysis, the extent of impedance decrease, the loss of pace capture, and achievement of transmurality post-ablation strongly favoured low-flow lesions, therefore supporting this approach to posterior LA ablation to prevent oesophageal damage.
Mechanical Displacement of the Oesophagus
Another potential intervention for oesophageal protection during LA ablation is actual mechanical oesophageal displacement. Proper identification of structures that lie in close proximity to the oesophagus is a critical step before mechanical displacement is considered. The oesophagus has been reported to be a highly mobile organ, without a serosal layer, and although fixed in the mediastinum (primarily in the pharynx and gastro-oesophageal junction), its position can be variable. Good et al., using barium paste and digital fluoroscopic imaging, reported that under conscious sedation the oesophagus shifts sideways at least 2 cm.18 Oesophageal movement varies along the LA surface, with a mean displacement of 2.0 ± 0.8 cm (range: 0.3–3.8 cm) in the superior portion of the oesophagus, 1.7 ± 0.8 cm (range: 0.1–3.5 m) at the mid-oesophagus, and 2.1 ± 1.2 cm (range: 0.1–4.5 cm) inferiorly. Accordingly, two small studies were published with the aim of addressing possible techniques for mechanical oesophageal displacement. Chugh et al. aimed to determine the feasibility of mechanical displacement through the help of an endoscope with a flexible tip in 12 patients during AF catheter ablation.44 In 9 of the 12 patients, this was a redo procedure performed because of an incomplete PVI and recurrent arrhythmia detection due to an unfavourable LA–oesophageal relationship during a prior ablation procedure. In 10 (83 %) of the patients, the oesophagus was displaced toward the left and right to a maximum of 2.4 and 2.1 cm, respectively. In two (22 %) of the nine patients in whom a prior procedure was unsuccessful due to rise in intraluminal oesophageal temperature, the displacement facilitated effective energy delivery at target site. The downside of this technique is that there is a theoretical concern that RF energy might be shifted from the ablation catheter to the endoscope. Therefore, RF ablation is only performed if the oesophagus remains in the same place after the probe has been removed. Using a different approach, Koruth et al. placed an endotracheal stylet within a chest tube to mechanically deviate the oesophagus in a cohort of 20 consecutive patients undergoing RFA or laser balloon for AF (there was no control group).45 Leftward oesophageal deviation of 2.8 ± 1.6 cm and rightward deviation of 2.8 ± 1.8 cm was observed. Temperature rose to more than 38.5°C in three (15 %) of the patients. There was no rise of temperature greater than 40°C. Post ablation, all of the patients had an upper gastrointestinal endoscopy to evaluate for ulcer formation. Of the 19 patients analysed, 18 (95 %) showed no ulceration from thermal injury. Overall, 12 patients (63 %) showed lesions that were classified as mild (9) and moderate (3) intensity oesophageal instrumentation-related trauma. During follow-up (mean 40.6 days), no patient developed dysphagia or gastrointestinal bleeding.
Transoesophageal echocardiogram (TOE) has also been used to mechanically displace the oesophagus during AF ablation procedures. Herwerg et al. used a TOE transducer to move the oesophagus away from the ablation sites in a total of three of six patients who underwent AF ablation via fluoroscopy.46 The increased distance created by the deflection allowed for a targeted RF energy at critical sites of the posterior LA wall. No patient showed evidence of oesophageal injury, and only one of the patients presented with mild pharyngeal oedema (Figure 5).
In a recent study, Parikh et al. evaluated the safety and efficacy of a novel mechanical oesophageal deviation tool, the EsoSure (NEScientific).47 In a multicenter trial, EsoSure was used to displace the oesophagus in 85 patients who underwent PVI and in whom the increase in oesophageal temperature limited the desired energy delivery at the posterior wall. All PVs were successfully isolated without a rise in oesophageal temperature (Figure 6). At 3-month follow-up, 7 % of patients reported self-limited dysphagia; no other major complications were seen. A randomised clinical trial testing a new investigational oesophageal deviation device by Reddy et al. (NCT01546168) was stopped due to lack of statistical significance after an interim analysis was performed. No difference was found between the use of this device for mechanical displacement and oesophageal temperature monitoring alone; results have yet to be published.
Mechanical displacement of the oesophagus appears promising and safe, and should allow for complete LA PVI, especially in patients with a thinner posterior atrial wall. Nevertheless, when performing displacement of the oesophagus, its complete anatomy should be delineated, as elegantly reported by Palanisway et al. Some probes have the disadvantage of not properly identifying the trailing edge of the oesophagus, which could lead to inadvertent injury.48 In addition, given the mobile nature of the oesophagus, trauma has been reported when a stiff stylet is used for mechanical displacement.45 Large prospective randomised clinical trials are still lacking, and therefore a more robust measure of efficacy and safety is yet to be determined.
Epicardial Balloon
Protection of the oesophagus can also be accomplished by inflating a balloon catheter (18 mm × 4 cm balloon dilation catheter; Meditech [Boston Scientific]) in the pericardial space. Buch et al. initially described this procedure during epicardial ventricular tachycardia ablation in a patient with repetitive drug refractory monomorphic ventricular tachycardia to mechanically distance the phrenic nerve.49 More recently, the same group described using this technique to protect the oesophagus and right phrenic nerve during AF ablation in a porcine model and in humans, thus allowing safe complete isolation of all four PVs.50,51 The distance between the oesophagus and posterior LA balloon inflation increased by 12.3 ± 4.0 mm, considerably attenuating the luminal oesophageal temperature increase during endocardial RFA (i.e. 6.1 °C versus 1.2 °C; p<0001).51 Similarly, after displacement of the right phrenic nerve with the intrapericardial balloon, nerve capture was abolished in 91 % of sites previously stimulated by pacing (Figure 7).51 This technique, while effective, does require epicardial access with its inherent risks.
Initiation of Proton Pump Inhibitors
Initial studies performed in canine models by Yokoyama et al. evaluated whether concomitant states of inflammation of the oesophagus, such as gastro-oesophageal reflux disease, may be a potential risk factor for oesophageal injury during LA ablation.52 Prior to this, Martinek et al. assessed the acute effect of RFA on distal oesophageal acidity.53 In this study, 31 patients with a diagnosis of paroxysmal AF were enrolled. Oesophagoscopy was used to evaluate for any injury prior to and after the ablation. No patient was on a PPI or had oesophageal acidity problems. Of 26 patients evaluated after ablation, 5 patients (19.2 %) demonstrated a significant pathological increase in the DeMeester acid reflux score evaluation. However, only one patient with asymptomatic reflux developed oesophageal ulceration. As a result of these findings, multiple centres are using PPIs or H2 blockers as a common clinical practice before and after the ablation procedure, with emphasis given to those with an history of gastro-oesophageal reflux disease.14,54 Gastric acid reflux is very common among patients undergoing RFA, and experimental studies have reported a correlation between reflux and the progression of oesophageal ulcers created by ablation (Figure 2).55 In patients undergoing lengthy ablation and/or multiple lines, assessment for previous history of reflux disease should be performed, and if positive or probable, post-ablation PPIs should be initiated.4 The efficacy of sucralfate (acid buffer) as a prophylactic treatment has not been tested extensively. Based on these findings, PPIs as a singular preventive treatment is recommended by the 2017 AF ablation consensus statement.32
Other Techniques
As an alternative strategy to RFA, the use of cryoablation for targeting AF triggers has been proposed in multiple studies. Ripley et al. applied cryotherapy in bovine oesophagus in vivo.56 Compared to the disruption of normal cellular architecture seen in RFA, macroscopic and histologic findings after cryotherapy showed no cellular change, chronic ulceration, or fistula formation. This is corroborated by an experimental study demonstrating that cryothermal ablation preserved the extracellular matrix and endothelial integrity.57 Clinically, in a prospective study by the OPIPAF group (Ostial Pulmonary vein Isolation in Paroxysmal Atrial Fibrillation), 70 patients underwent cryotherapy as the preferred treatment for PVI.58 No PV stenosis or oesophageal injury was detected during follow-up of 33 ± 15 months post ablation. Regarding efficacy, however, 49 % of the patients achieved complete initial success, with 11 % (8) of patients showing sporadic episodes of AF post ablation.
Initially it was thought that cryotherapy would not be associated with PV stenosis, oesophageal perforation, or thromboembolic events. However, in a well-reported systematic review by Andrade et al., the procedure was found not to be free of serious complications, the most common of which was phrenic nerve paralysis, with an overall incidence of 6.38 % (86 of 1349 procedures) despite the high acute procedural success rate (91.67 % complete PVI in 19 studies and 94.85 % complete PVI of targeted veins in 18 studies).59 Also seen were pericardial effusions, which occurred in 1.46 % of cases, and thromboembolic complications in 0.57 % of cases. However, no cases of left atrio-oesophageal fistulas were reported. Nonetheless, Ahmed et al., reported oesophageal ulcerations in 17 % of the 35 % of patients in whom endoscopic evaluation was performed after CB ablation.60 More recent evidence has been published verifying that CB ablation is not free of oesophageal damage. John et al. reported that the incidence of atrio-oesophageal fistulas with CB ablation is similar to that of RF ablation (<1 in 10,000).12,61 Of note, they noted that there was a particular relationship between the left inferior pulmonary vein ablation and fistula formation. Prolonged CB inflation times were noted in the fistula occurrences. The authors proposed that real-time monitoring for temperature decline in the oesophagus should quickly prompt limiting energy delivery through this method.
Oesophagus Cooling Systems
Oesophageal cooling systems are used to mitigate conductive heating to the oesophagus (therefore preventing ulceration due to thermal damage). Arruda et al. conducted an experimental model of an oesophagus cooling system, and the results were promising.62 By circulating fluid constantly at 5 or 10 °C and using a compliant system with adjustable circulating volume to avoid displacement of the oesophagus, high power (45 W) could be achieved. In a recent randomised controlled study, 100 patients who underwent RF ablation of AF were assigned to either oesophageal cooling with 5 mL of iced water or ablation without oesophageal cooling.63 The authors found that oesophageal lesions assessed by endoscopy might be less severe in the cooling group; however, no difference in the incidence was reported (20 and 22 %, respectively). Studies in larger populations are still needed to evaluate the true effectiveness of this method, and the practice is not currently recommended as routine according to the most recent consensus statement on AF ablation.32
Early Identification of a Suspected Atrio-oesophageal Injury
In a recently published article by Kapur et al, a stepwise algorithm to an early detection of a potential atrio-oesophageal injury is described. A non-emergent evaluation that includes performing an upper endoscopy during the 72 hours following the procedure should be considered in patients with extreme temperature registered at the oesophageal probe during the procedure, have symptoms immediately after ablation and have a pre-existing oesophageal pathology.64 On the contrary, an emergent evaluation should be performed in all patients who present during the 6 weeks following the ablation with symptoms such as chest pain, fever or gastrointestinal or neurological symptoms along with the abovementioned characteristics for a non-emergent procedure. This emergent evaluation should include blood laboratory, transthoracic cardiac echocardiogram and chest CT with IV contrast.64 Despite the low incidence of fistula formation, early diagnosis and rapid surgical treatment are cornerstone in the management of this complication.
Conclusion
Though rare, serious oesophageal injury during PVI and posterior wall ablation is still a major concern among electrophysiologists, particularly if left untreated, with a mortality rate of up to 100 % associated with atrio-oesophageal fistulas. In our practice and experience, we rely on a stepwise approach to prevent these potential complications. Several techniques have been described to prevent such injuries. We suggest obtaining pre- and intra-procedural oesophageal imaging to provide valuable information for ablation, given the high variability of oesophageal anatomy among patients. Despite its controversy, our group encourages the routine use of oesophageal intraluminal temperature monitoring probes during AF ablation. Mechanical displacement of the oesophagus appears feasible, safe and efficacious; nevertheless, randomised data are missing to evaluate the different tools available. Consistently in our procedures, when performing ablation lesions to the posterior wall, we prefer to use low irrigation parameters as this technique has been shown to be effective and seems to be a promising way to avoid oesophageal damage. We do not routinely use cryothermal energy ablation; however, it has been shown to have a lower incidence of oesophageal injury,64 though the data is not without debate and efficacy may be less than RFA. Given the low incidence of clinically significant events, such as atrio-oesophageal fistula, the development of strategies for the prevention of these potential complications is limited. As discussed in this review, the use of different surrogates, such as variation in oesophageal luminal temperature, remains controversial and more studies are needed to evaluate oesophageal injury prevention systems.
Clinical Perspective
- Oesophageal injury prevention has become a major concern in the field of electrophysiology.
- Oesophageal wall injury varies from erythema to ulceration, is common and has been described by endoscopy in up to 47 % of patients following pulmonary vein isolation (PVI).
- Assessment of the anatomic relationship between the LA and the oesophagus prior to the procedure is of major importance.
- Different techniques, including mechanical displacement of the oesophagus, intraluminal temperature monitoring, and adjusting to low irrigation parameters are options to be considered when performing lesions to the posterior wall in an attempt to avoid any potential injury.
- Emergent diagnostic procedures should be performed in patients with high suspicion of atrio-oesophageal fistula.