Recurrent episodes of ventricular tachycardia (VT) in patients with structural heart disease are associated with increased mortality and morbidity, despite the life-saving benefits of implantable cardiac defibrillators (ICDs).1,2 Because ICD therapies are abortive and do not alter the underlying arrhythmogenic substrate, their reduction becomes important, especially as recurrent shocks can cause increased myocardial damage and stunning, despite the conversion of VT/VF.3,4 Antiarrhythmic drugs can reduce the number of ICD therapies, but their long-term use is hindered by side-effects, especially with the most effective agent, amiodarone.5 In the absence of revolutionary drugs or antifibrotic regenerative therapies, catheter ablation has emerged as a potential therapeutic option either for primary or secondary prevention of these arrhythmias. This is particularly relevant to post-MI cases where the substrate is well defined. However, the outcomes of catheter ablation of VT in structural heart disease remain unsatisfactory in comparison with other electrophysiological procedures; initial first time success rates are only 50–70 % in most large series versus 80–90 % in normal heart VT.6 The disappointing efficacy of VT ablation in structural heart disease is multifactorial, relating to both our inability to fully delineate the substrate’s fixed and functional electrophysiology determining VT initiation and maintenance, as well as the delivery of adequate lesion sets to the critical arrhythmogenic sites.
Mapping during VT
There have been rapid advances in the field of ventricular arrhythmia therapy over the past two decades following the seminal studies characterising post-infarction VT substrates during cardiac surgery.7–10 Activation mapping is now utilised to locate the point of the earliest activation of tachycardia, with entrainment subsequently performed to not only confirm re-entry as the arrhythmia mechanism but to allow for accurate delineation of the critical components of a circuit.11 The main target for ablation being the central isthmus, represented by mid-diastolic potentials during VT and specific entrainment criteria.12 The entrainment of VT in the right ventricle of a patient with arrhythmogenic right ventricular cardiomyopathy is illustrated in Figure 1.
Electro-anatomical mapping and advances in catheter technology have made VT ablation procedures routine in tertiary centres, particularly in post-infarction VT where arrhythmias are more amenable to these activation and entrainment mapping strategies. Indeed, elegant mechanistic studies have identified the role of border zone ultrastructure, scar geometry and anisotropy in determining wavefront curvature and functional block critical to initiate re-entry.13 Ciaccio et al. showed that infarct scar geometric features can be utilised to localise VT isthmuses, and sites of slow stable conduction lie in close proximity to sites of functional block.14 These principles have been applied clinically in post-infarction VT and recently utilised to define pro-arrhythmic areas based on electrogram morphology and stability; for example, Shannon entropy.15,16 However, in non-ischaemic cardiomyopathy, specific features of the substrate (e.g., myocyte disarray promoting anisotropy in hypertrophic cardiomyopathy or disruption of myocyte electro-mechanical coupling by desmosomal mutations in arrhythmogenic cardiomyopathy) create functional changes in the tissue to promote conduction block and re-entry. Furthermore, the scar is often very diffuse, making simple delineation of border zones seen in post-infarction VT challenging to define for substrate modification in these disorders.
Therefore, functional changes independent of fibrosis might play an important role in ventricular arrhythmogenesis. This means that ablation relying on identifying fibrotic regions as potential VT isthmuses is probably inadequate, especially since it is assumed that the mechanism of VT involves a critical isthmus, but it is also possible that micro re-entry in an adjacent site is the primary driver with a bystander isthmus.17 Such sites will elude conventional ablation catheter bipolar mapping due to lack of resolution, but could be defined in tachycardia with higher-density mapping catheters (e.g., PentaRay or Orion basket); the functional and structural features of these sites can be dissected. This would explain the lower success rates of ablation in dilated cardiomyopathy versus post-MI VT, as the mechanism has not been defined or the circuit eludes conventional ablation catheter mapping.18–20
Therefore, despite our ability to identify post-MI VT isthmuses with a view to ablation in stable circuits, there remain significant knowledge gaps in predicting the probability of VT and their anatomical location in individual cases, especially non-ischaemic cardiomyopathies, and the planning of the substrate modification required.
As a result, extensive endo-epicardial ablation and ‘scar homogenis ation’ are frequently performed to modify potentiallyarrhythmogenic sites with limited functional analysis.21 This risks unnecessary myocardial damage and pro-arrhythmia.22 Furthermore, in cardiomyopathy patients, diffuse areas of epicardial fibrosis act as potential re-entry sites, but the arrhythmias are often unmappable because they are haemodynamically unstable or not sustained.23 A deeper understanding of mechanisms of VT in these cases (i.e., micro re-entry, functional block leading to isthmus formation, repetitive Purkinje activity, the interplay between structural inhomogeneities and dynamic conduction–repolarisation interactions) is required to optimise therapeutic targeting and risk stratification in VT.
A major limitation of mapping during VT is haemodynamic intolerance of the arrhythmia, with as few as 10 % of arrhythmias induced being stable.24 Advances in interventional cardiology have led to smaller infarct sizes and VTs with shorter cycle lengths accounting for the decreasing use of mapping during tachycardia due to their haemodynamic instability or transient nature.25 The obstacle posed by unstable VTs could be addressed using haemodynamic support devices. Percutaneous left ventricular assist devices allow more detailed and prolonged mapping of unstable VTs, with more VTs terminated by ablation, although no impact on the inducibility of VT at the end of procedures is evident.26 Whether these disappointing results are due to selection bias needs to be analysed in further trails.27 Extracorporeal membrane oxygenation has also been studied in this context. Its use allows for mapping, ablation and subsequent non-inducibility of VTs that were previously inducible at the end of substrate-based approaches. In this study, the acute procedural success, in terms of non-inducibility, was associated with better longterm outcomes. The approach had low complication rates and could be increasingly utilised in the future. 28 However, prospective studies are lacking and will be necessary for rigorous evaluation of the technique.
On a practical level, although entrainment mapping is seen by many as the gold standard for the interrogation of a re-entrant circuit, it also suffers limitations that stretch beyond the afore-mentioned haemodynamic issues. Problems include inability to capture or having to increase pacing outputs, which increase the volume of captured tissue leading to inaccuracies in defining the exact site of the isthmus, as the captured area can be over 2 cm away. Even if consistent capture is achieved, oscillations in the tachycardia cycle length can introduce errors, resulting in misleading post-pacing intervals or local tissue properties causing latency and long return cycle lengths, appearing as bystander sites. These confounding issues might only become apparent after ablation has not terminated the tachycardia. Finally, due to the muscular bundles that form the isthmus being only a few hundred microns in diameter, the diastolic component can be very low in amplitude and difficult to detect, especially if the noise levels in the catheter laboratory are high and mapping system filters saturated at specific frequencies.29
Substrate Mapping
Due to these limitations, and the fact that patients can have multiple haemodynamically unstable or non-sustained VT, substrate mapping and ablation have gained popularity. During sinus rhythm mapping, low-amplitude, fractionated electrograms and late potentials associated with surviving bundles of myocardial tissue surrounded by fibrous tissue are identified.30,31 Early work employing subendocardial surgical resection of these areas eliminated approximately 50 % of the abnormal signals and reduced VT recurrence.32 Homogenisation of scars, involving ablation of these abnormal potentials within scars, reduces VT recurrence in patients with ischaemic cardiomyopathy.21 Translation of these results to non-ischaemic cardiomyopathy is difficult, with outcome measures less impressive than with ischaemic cohorts.22 The extensive ablation of late potentials in a patient with arrhythmogenic right ventricular cardiomyopathy is illustrated in Figure 2.
Extensive ablation of local abnormal ventricular activities has also been demonstrated to significantly reduce VT recurrences in secondary prevention cases, but extensive ablation is required with long procedure times of up to 186 +/–78 minutes. This highlights the question of how much ablation is truly required to prevent recurrences.33 As such, there is no universal agreement on optimal ablation strategy for scar substrate. Randomised trials examining substrate-based ablation exist in the form of the ablation of clinical ventricular tachycardia versus addition of substrate ablation on the long term success rate of VT ablation (VISTA) and the substrate mapping and ablation in sinus rhythm to halt ventricular tachycardia (SMASH-VT) trials. The VISTA trial demonstrated that an extensive substrate-based approach was superior to a more focused activation mapping strategy. For obvious reasons, only tolerated VT was included in the activation mapping arm, and whether these results can be extrapolated to the wider VT cohort of patients is unclear.34 The randomised, primary-prevention trial, SMASH VT, showed a reduction in VT events using a substrate-based approach.35 The positive results of this trial, which achieved a 70 % reduction in arrhythmic events at 2 years, has not been replicated.
There are many reasons that could explain why the results have been disappointing. Voltage mapping using standard parameters of >1.5 mV as normal tissue and <0.5 mV as scar tissue might lead to underestimation of the heterogeneity of the tissue mapped.36 The use of bipolar voltage to locate islands of surviving myocardium that could form conducting channels supporting VT is limited by the current technologies employed, primarily 3.5-mm bipolar ablation catheters. Using smaller electrode sizes with closer bipolar spacing to minimise far-field potentials can increase resolution, and has been to shown delineate areas of surviving myocardium that were labelled as inert scars on maps produced with larger electrodes (e.g., using multipolar PentaRay and duodecapolar catheters).37–39 Electrode spacing is not the only potential source of error when attempting to delineate scars. The angle at which the catheter is placed on the muscle can lead to falsely low bipolar voltage if it too steep, as the electrodes are activated simultaneously and signal cancellation occurs.40
Wavefront direction is another source of error, with late potentials present during apical pacing disappearing during ectopic beats.36 This phenomenon is also seen when comparing maps created during sinus rhythm and during right ventricle pacing. Sinus rhythm maps show larger scar areas with fewer late potentials.41 Similarly, differential pacing sites during mapping impact voltage maps created. With the characterisation of scars being different, especially in septal locations and areas of low density, the sensitivity of voltage maps could be improved by using separate pacing locations during mapping.42 Furthermore, isthmuses present during VT might be absent when mapping in sinus rhythm. The concept of block being functional rather than anatomical has been shown in computer models of VT and in clinical studies.43,44 In essence, dynamic changes in conduction repolarisation lead to transient lines of block, which are cycle-length dependent, creating the opportunity of re-entry to develop at a site. Mechanistically, tissue susceptibility to re-entry depends on the spatial interaction between refractoriness and conduction dynamics, as re-entry requires that a wavefront of excitation finds electricallyexcitable tissue always ahead of it. Favourable conditions for re-entry might be met when conduction velocity is slowed by a premature beat and where short repolarisation allows the tissue to regain excitability, therefore potentially enabling the establishment of a re-entrant circuit.45 A metric to quantify tissue susceptibility to re-entry and predict critical sites for VT initiation based on this principle has recently been proposed.46
Localising a VT exit site is often attempted during ablation using the 12-lead electrocardiogram (ECG). These sites can be paced during sinus rhythm and compared morphologically to 12-lead ECGs, with most modern mapping systems containing software to produce a degree of match between the two. Due to the prevalence of ICDs in the patient cohort, a 12-lead ECG of the clinical VT is rarely captured, and the cycle length of the tachycardia stored on an ICD is often used to identify whether an induced VT is likely to be clinical. Despite the lack of a preprocedural ECG, VT induced during the case can be used to guide the operator to potential areas of interest. In idiopathic VT, the 12-lead ECG can localise anatomical regions where a VT exit site is most likely to be present. While similar algorithms have been used with some success in patients with structurally-abnormal hearts,47 the applicability of facets of these algorithms is questionable. In an invasive non-contact mapping study, the use of concordance in the precordial leads was not found to be useful in infarct-related VT.48 Furthermore, algorithms to assess for epicardial exit sites, which would be useful in preprocedural planning, have proved inaccurate.49 Pacing in sinus rhythm from sites of concealed entrainment produces unmatched pace maps in just under one-third of cases.50 Proposed mechanisms underlying the limitations of pace mapping include differential areas of block present during sinus rhythm and VT resulting in different QRS morphologies, and different pacing outputs resulting in divergent myocardial capture.51 Despite these issues, the utilisation of pace mapping to locate, and subsequently ablate, scar areas with multiple exit sites has been shown to improve VT-free survival in a single-centre study;52 the implication being the potential to deliver more targeted ablation lesions in regions more likely to support VT.
Epicardial Access
Studies investigating non-ischaemic cardiomyopathy have demonstrated a greater proportion of potentially-arrhythmogenic substrate in the epicardium compared to the endocardium.53,54 These studies utilised percutaneous epicardial access for mapping, with this technique first described in patients with Chagas disease.55 Cano et al. found that epicardial low-voltage areas were more prevalent and larger in the epicardium compared to the endocardium in nonischaemic cardiomyopathy.54 In ischaemic cardiomyopathy, its utility is less convincing, with an endocardial predilection for scars in these cohorts. However, when initial endocardial ablation has failed, a combined approach in redo procedures might produce positive results.56 Damage to subdiaphragmatic organs during puncture can occur, and haemorrhage from diaphragmatic vessels has been reported. Pericardial effusion is common; it occurs due to damage caused to the myocardium by ablation and puncture of the right ventricle or coronary arteries. The latter of these also needs to be considered during ablation, with imaging utilised to ensure ablation is remote from the arteries.57 Despite these potential risks, it has proved to be a safe procedure with low complication rates.58 In summary, although current practice varies in different centres, an epicardial approach is usually performed when there has been a failed endocardial approach or the underlying substrate is likely to be epicardial. The latter is delineated based on aetiology and potentially preoperative scar imaging.59
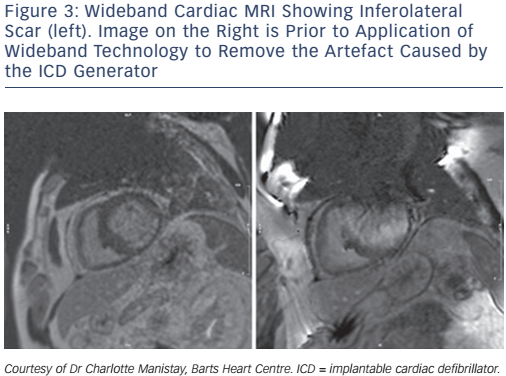
Complications
Major complications occur in approximately 8–10 % of VT ablation procedures,60 with lower mortality. The three main complications are vascular injury, stroke and cardiac tamponade. In a large, single-centre study of both idiopathic VT and structurally-abnormal heart VT, accesssite vascular injury was the most frequent complication at 3.6 %, with stroke and tamponade both <1 %.61 No procedural deaths occurred in this study. The incidence of vascular complication might be higher in ischaemic heart disease cohorts, and although the incidence of stroke is low, the use of irrigated catheters is also purported to reduce the risk of stroke further by preventing coagulum formation on catheter tips.62 Complications can perhaps be further minimised with the use of a lower-power output of 30–40 watts during ablation.61 Anticoagulation during ablation is achieved with intravenous heparin, and post-ablation anticoagulation is recommended for 6–12 weeks with either aspirin or warfarin.63
Endpoints for VT Ablation
Traditional focus is on the labelling of clinical and non-clinical VTs occurring during ablation. Clinical VT being denoted as such if it is similar to the 12-lead ECG, the cycle length recorded from an ICD or occurs spontaneously during mapping. However, arrhythmias labelled as non-clinical might in fact occur spontaneously in the out-of-hospital setting.12 Studies have differed in their approaches to these distinct re-entrant circuits. Using programmed electrical stimulation at the end of a procedure, some focus only on the clinical VT and others have included non-clinical VTs for their non-inducibility endpoints. Guideline consensus states that non-inducibility of clinical VT in response to programmed electrical stimulation should be considered the minimum endpoint after ablation.63 Della Bella et al. found that non-inducibility of all VTs was associated with significantly lower cardiac mortality.64 Others have shown inducibility of nonclinical VT to be associated with recurrence.65,66 In contrast, a recent pooled analysis of studies showed that non-inducibility of VT was not associated with lower recurrence.67 With no available studies specifically designed to address the question of optimal endpoints, it is difficult to produce standardised outcome measures.
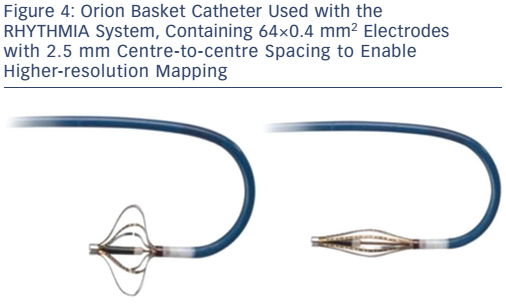
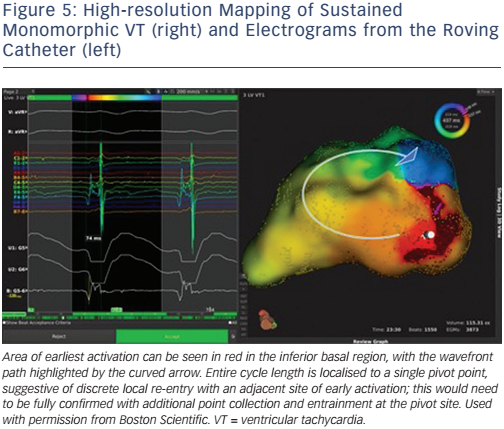
The recent increased use of substrate homogenisation necessitates a standardised method for assessing whether all targeted late potentials have been successfully ablated.59 Scar remapping is one suggested method of assessment, and with more expedient mapping, technologies might become more commonplace.68 Lack of capture with high-output pacing has been used in addition to late potential abolition as an endpoint. This has been employed particularly when late potentials are still recorded, despite extensive delivery of radiofrequency energy.21
Future Directions
Cardiac MRI Delineation of Scar and Pro-Arrhythmic Circuits
Cardiac MR imaging of scars shows promise as an adjunct to traditional electrophysiologically-based mapping. Gadolinium late enhancement demarcates extracellular space and is a well-validated surrogate marker of dense fibrosis. It can delineate heterogeneous tissue within scars that could form the isthmus of a re-entrant circuit.69
Despite the potential utility of cardiac MRI to identify potential ablation targets, its use remains limited. The main problem being the artefact caused by ICD generators and leads in many VT patients. A novel wideband technique enables optimised scar evaluation in patients with ICDs without any adverse sequelae (Figure 3). The acquired pixel spatial resolution of cardiac MRI using wide-band late enhancement at our centre is fixed by the sequence parameters at 1.4×1.4×4 mm (contiguous segmented 4-mm slices throughout the ventricles). This represents a sample of 8 µg of myocardium using non-interpolated data. It compares favourably to previously-published data for scar imaging by MRI, and is similar to resolution of voltage maps in vivo (3–5 mm). Crucially, MRI-derived scar analysis can also determine scar transmurality with distance from endo/epicardial borders. A transmural scar of >75 % corresponds to areas of slow conduction, known to be a necessary component for a re-entrant circuit, and scars >25 % of left ventricle wall thickness is necessary for critical sites involved in the maintenance of VT.70 Recent work has highlighted the capacity of navigator-gated inversion recovery 3D sequencing to correctly identify conducting channels when compared to electro-anatomical maps. The technique correlates well with electro-anatomical maps, and no significant deviation is seen in lead parameters.71 The availability of the integration of MRI scar and channel maps into available electroanatomical mapping software could provide valuable information to electrophysiologists during catheter ablation. More studies need to be conducted to evaluate both the validity and efficacy of this approach. This is especially relevant when one considers the resolution of cardiac MRI relative to that of contact mapping catheters and the size of the channels in question, which might be only be <1 mm in diameter. Cardiac MRI using 1.5 tesla magnets has a resolution of 4 mm, whereas 2-mm (1-mm spacing), high-density EP catheters, which take into account stability and electrode density, can resolve up to 2 mm of tissue and recognise very low amplitude signals in diffuse scars beyond the resolution of gadolinium.72 This is because gadolinium uptake is measured relative to ‘normal’ segments, and thus could miss diffuse fibrosis akin to balanced ischaemia being reported as ‘normal perfusion’ on nuclear imaging.
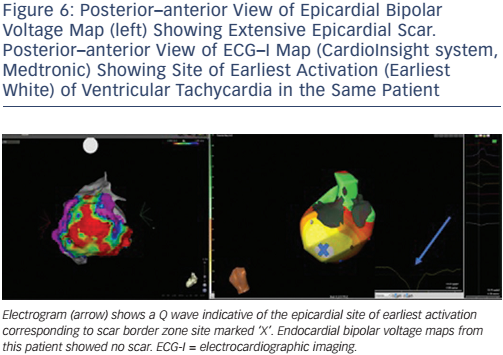
Multielectrode High-Resolution Mapping
The limitations of current mapping systems have demanded the development of new technologies that achieve higher temporal and spatial resolution. This is made possible by using smaller electrodes with closer spacing, automated software allowing rapid data collection and accurate time annotation of electrograms with multiple components. The RHYTHMIA high-density mapping system, utilising a 64-electrode, high-density mapping Orion basket catheter, has led to important insights into the mechanisms of VT (Figures 4 & 5). A porcine study confirmed that in mapped sustained VT, portions of block in a re-entrant circuit was functional rather than anatomical.73 This finding confirms previous work stated above72, and has been confirmed in a further study.
Entrainment mapping was also employed in this study, and areas where criteria for entrainment were met were associated sites distinct from the re-entrant circuit. The length of isthmuses was also overestimated by entrainment.73 Although allowing for faster mapping, this system does not solve the problem posed by haemodynamically-unstable rhythms, as sequential mapping in tachycardia is still required. It could however improve outcomes in tolerated VT. With regards to substrate mapping, the RHYTHMIA system, in conjunction with a basket, shows better correlation with MRI scar maps than standard catheter mapping.74
Reported human studies are thus far limited, with most studies examining the atrial substrate. In one series, the system was able to successfully map 24 of 28 atrial tachycardias that were incompletely mapped during the prior procedures employing standard techniques, and 22 were successfully terminated.75 The safety and efficacy of the procedure in ventricular arrhythmia have been reported in one study. Findings were positive, showing no complications, consistent recording of abnormal electrograms and good medium-term outcomes for freedom from VT.76
Other technologies, such as ripple mapping, might also help solve problems posed by current mapping techniques. The system displays electrogram components as a dynamic bar that protrudes from standard geometry. It has been shown to correctly identify conducting channels in scarred myocardium, and has been used in VT ablation with successful preliminary results.77,78 These newer mapping systems could be further integrated in future with technologies, such as intracardiac echocardiography, to allow improved visualisation of ventricular anatomy.79 Additionally, this technology has been shown to accurately identify wall motion abnormalities and corresponding scars,80 thus potentially aiding in the localisation of the arrhythmogenic substrate, and can aid ablation in regions of the heart that are troublesome to target.81
Electrocardiographic Imaging
The global changes in electrophysiological parameters over both ventricles are not easily accessible using conventional sequential mapping techniques, and thus information is lost. This is especially critical to modelling approaches attempting to predict arrhythmia, which then have to rely on structural surrogates, such as gadolinium uptake and diffusion on MRI, to estimate conductivity parameters as opposed to global electrophysiological data. The ECG Imaging (ECG-I) system, developed by Yoram Rudy, utilises inverse solution mathematics to generate a unipolar electrogram map derived from high-density surface ECG jacket recording, which is projected onto the patient’s CT-derived cardiac geometry. It has been validated in canine models, patients undergoing cardiac surgery and ablation of focal ventricular ectopy.82–89
ECG-I has the potential to map arrhythmias using a single cardiac cycle, and thus could be relevant in the mapping of unstable VT (Figure 6). When assessing ECG-I data in patients with prior MI, a good correlation between scars on MRI and low-amplitude virtual electrograms was demonstrated. Fractionated potentials also had good correlation with sites of fibrosis on MRI, albeit less so than for low-amplitude electrograms. This feature could be attributable to the false identification of epicardial fat as scars on MRI. Late potentials can also be seen within scarred myocardium. During sinus rhythm mapping, these scarred areas alter the activation wavefront and form areas of conduction block. This information could be used to limit ablation to specific pre-identified regions when a substrate-mapping approach is employed.90
The system has also studied post-MI VT. Activation time was used to map the re-entrant circuits, which were correctly mapped to areas of scar. Predictions of site of onset were accurate for distinguishing between epicardial, intramural and endocardial locations utilising electrogram morphology; that is, pure Q waves representing true epicardial sites. This latter point highlights an important facet for clinical use. The prediction of the necessity of epicardial access could significantly shorten procedure times and enable a more focused ablation strategy.91 Additionally, given that many VTs cannot be sequentially mapped, knowledge of the exit site would be invaluable for the clinician during ablation. Current validation studies show an accuracy for mapping the site of origin of 4–6 mm.92 This degree of resolution might not be sufficient to guide ablation, but could enable expedited pace mapping of exit sites. Whether these potential applications would improve outcomes need to be tested in large trials, although procedure planning, workflow and catheter laboratory time might be reduced.
Conclusions
Advances in high-density and non-invasive mapping technologies are enabling far more detailed interrogation of myocardial substrates than previously possible, coupled with major technological developments in imaging. This means that we can now study potential circuits and plan procedures in a more informed manner.93 The key challenges of mapping unstable VTs remain to be fully addressed, as well as optimising substrate-based approaches to enable more targeted, less extensive ablation, if this is possible. This will require deeper understanding of the dynamic behaviour of the tissue critical to facilitate re-entry, with the ultimate goal of undertaking more personalised and refined ablation strategies.