Pioneering electrophysiology studies in the 1990s defined the anatomical boundaries of typical atrial flutter, identified regions for effective catheter ablation of this arrhythmia and described procedural endpoints to minimise recurrences after ablation. Activation and entrainment mapping demonstrated that typical flutter arises from reentry around the tricuspid annulus.1 Criteria to confirm bidirectional conduction block in the cavotricuspid isthmus (CTI) became standard procedural endpoints and improved freedom from long-term recurrences. Principles learned from these studies of typical flutter have permitted better understanding of more complex atrial arrhythmias. Through the use of multielectrode catheters and electroanatomical mapping, as well as entrainment, atypical atrial flutters and atrial tachycardias (ATs) of various mechanisms have been defined. The demonstration of bidirectional conduction block across linear lesions has been adopted as an important endpoint in ablating atypical flutters in both atria. The purpose of this review is to summarise current concepts of atypical flutters and ATs which are not dependent on the CTI.
Focal Atrial Tachycardias
Focal ATs are defined as arrhythmias that arise from a circumscribed site of early activation and propagate to the atria in a centrifugal pattern. These types of AT can occur in patients with structurally normal atria as well as those with structural heart disease. The mechanisms that give rise to focal ATs are triggered activity and automaticity. A consistent feature of focal ATs is sensitivity to adenosine, which terminates tachycardias due to triggered activity and transiently suppresses tachycardias related to enhanced automaticity but does not affect reentrant ATs (Figure 1).2
Focal ATs can arise from anywhere in the atria, but typically cluster in particular anatomical distributions. Common locations in the right atrium (RA) include the tricuspid annulus, the crista terminalis and coronary sinus ostium. Para-Hisian ATs demonstrate properties consistent with tachycardias from other sites around the tricuspid annulus and are thus best considered to be a subset of annular ATs.3,4 In the LA, common sites include the pulmonary veins and the mitral annulus, particularly the left aortomitral continuity. Other locations include the proximal coronary sinus, the atrial appendages and the septum.
Electrocardiographic Features
The P-wave morphology of focal ATs depends on the site of origin and conduction characteristics of the atria (Figure 2). In contrast to macro-reentrant ATs, focal ATs are more likely to demonstrate an isoelectric interval on the surface ECG.5 However, the presence of an isoelectric interval is only modestly sensitive and specific for a focal mechanism.6,7 Some focal ATs do not demonstrate isoelectric intervals if the atrial rate is very rapid and conduction times across the atria are slow relative to the tachycardia rate. Conversely, some macro-reentrant ATs demonstrate isoelectric intervals when conduction proceeds through a narrow isthmus that does not generate sufficient voltage to produce a deflection on the surface ECG.
Focal ATs that originate from the RA often have negative deflections in lead V1, particularly if they arise from more anterior structures such as the tricuspid annulus. Focal ATs from other RA sites, such as the crista terminalis, may generate biphasic positive/negative or even positive P-waves.8 The P-wave axis reflects the tachycardia origin in the inferior or superior part of the RA.9 Thus, ATs that originate from the high crista terminalis, high RA and appendage generate positive P-waves in the inferior leads. Para-Hisian ATs have narrower P-wave durations compared with sinus rhythm because centrifugal activation from a septal focus leads to parallel activation of the RA and LA; the inferior leads may be either negative, positive or biphasic; and lead V1 is often biphasic but sometimes has isoelectric components.3
Focal ATs that originate from the LA usually demonstrate positive deflections in V1 and across the precordial leads because the LA is attitudinally posterior in the chest.9 A negative or isoelectric P-wave in lead I was found to be 100% specific but not sensitive for an LA focus.10 ATs arising from the right pulmonary veins show monophasic P-waves, whereas those from the left pulmonary veins are broader and have notching in V1 and the inferior leads. Tachycardias arising from the mitral annulus have diverse P-wave morphologies depending on their origin in the annulus; they usually show initial small negative components followed by positive deflections.8 ATs that arise from the interatrial septum also characteristically demonstrate narrow P-waves.
It should be noted that P-wave morphologies of focal ATs may be altered in the presence of extensive prior ablation, atrial surgery or significant atrial myopathy.
Mapping and Ablation
Electroanatomical mapping has proved useful in identifying the origin of focal tachycardias, demonstrating centrifugal activation from a discrete location. The site of earliest activation should precede the P-wave onset by at least 30 msec, and the unipolar electrogram demonstrates a QS configuration with a steep negative deflection preceding the P-wave (Figure 1B). Ablation can be accomplished with radiofrequency (RF) energy using solid 4-mm electrodes, irrigated RF or large tip electrodes. In the LA, ablation with an irrigated catheter is preferred to minimise the likelihood of thrombus formation. Cryoablation is useful in regions near critical structures, such as near the phrenic nerve or atrioventricular (AV) node.
Successful ablation of ATs that demonstrate early RA activation near the His bundle require an understanding of the septal anatomy and the relationship among the tricuspid annulus, mitral annulus and aortic root. Para-Hisian ATs are commonly ablated in the non-coronary cusp of the aortic valve. Ablation in this location minimises the risk of AV block and it is often effective even if activation is slightly later than the para-Hisian site in the RA (Figure 3).11–13 ATs that arise from the septal mitral annulus also demonstrate early RA activation in the His bundle region, but left atrial (LA) mapping identifies even earlier activation and localises the site of effective ablation.11,14 A step-wise approach may be employed to mapping para-Hisian ATs: Careful mapping of the tricuspid annulus and RA septum may identify early activation at some distance (>1 cm) from the His bundle, where ablation can be successfully and safely performed. When the His bundle region demonstrates earliest RA activation, mapping and ablation of the non-coronary cusp should be attempted. If this is not successful, mapping of the LA septum should be considered. In some patients, mapping of the LA may precede the non-coronary cusp based on suspected site of origin and perceived risk of aortic access. Finally, ablation can also be performed in a true para-Hisian location in the RA, where cryoablation may minimise the chances of irreversible AV block.
Macroreentrant Atrial Tachycardias
Macro-reentrant ATs other than typical CTI-dependent atrial flutter often occur in patients with atrial disease, such as those with cardiomyopathies, prior atrial ablation or prior cardiac surgery. In the present era of ablation for AF, macro-reentrant ATs most commonly occur after linear ablation in the LA or RA. The incidence of AT after AF ablation varies, depending on the techniques and lesion sets employed to treat AF. Subsequent ATs are less common after pulmonary vein isolation (approximately 5% incidence) compared with patients treated with linear lesions and ablation of complex electrograms (about 25%).15–18 While macro-reentrant arrhythmias are the most common form of AT following AF ablation, focal arrhythmias also occur in a minority of such patients.
A hallmark of macroreentry is the ability to entrain the tachycardia with fusion; this property is inconsistent with a truly focal source. Another feature of macroreentry is identification of activation throughout the tachycardia cycle length with adjacent zones of ‘early’ and ‘late’ activation relative to a fiducial point. Macro-reentrant tachycardias are consistently insensitive to adenosine, which results in AV block but does not interrupt the tachycardia (Figure 1C).2 The locations and dimensions of macro-reentrant circuits in the atria vary considerably, but several common variants are recognised, which are described below.
Electrocardiographic Features
The flutter wave morphologies in macro-reentrant ATs are highly variable and there are few invariable features that identify specific reentrant circuits. Atypical flutters from the RA often demonstrate predominantly negative deflections in V1. However, other RA tachycardias, such as counterclockwise CTI-dependent atrial flutter, have positive deflections in V1, typically preceded by an isoelectric or negative component. Atypical flutters from the LA often show broad positive deflections in V1 but may show initial negative followed by positive deflections. The limb and precordial leads in LA flutters often show very low-amplitude signals, particularly in patients who had prior ablation. These generalisations are often violated, as the extent and distribution of atrial scar influence the resulting flutter wave morphology.
Principles of Mapping and Ablation of Macroreentrant Tachycardias
When a macro-reentrant circuit is suspected, it is important to verify that the entire cycle length is accounted for in the electroanatomical map. Missing segments of the cycle length could be related to areas of slow conduction that were not annotated on the map. Such areas typically exhibit low-amplitude and fractionated electrograms, with amplitudes as low as 0.05 mV or less.19 Alternatively, the inability to annotate all segments of the tachycardia cycle length might indicate the presence of a focal AT. Fractionated and prolonged electrograms pose a challenge to activation mapping, in that it is difficult to assign a single activation time to an electrogram with multiple components. Newer display and annotation techniques can account for multiple deflections in complex electrograms by visually representing multiple deflections. One such technique, known as ripple mapping, displays dynamic bars that extend from the surface of the map to indicate changes in the electrogram voltage.20 Another technique uses a window of interest to highlight regions that activate at particular times. These techniques can identify zones of slow conduction that might not be recognised by annotating single activation times.21
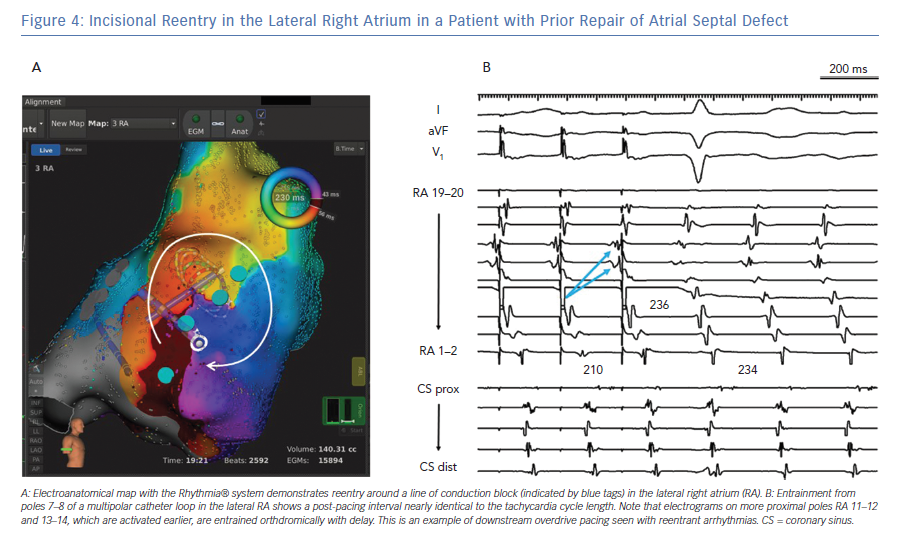
Entrainment confirms that a particular site participates in the tachycardia circuit when the post-pacing interval is within 30 msec of the tachycardia cycle length. In the case of macro-reentrant tachycardias, this criterion is fulfilled in two or more different segments of the atrium. For example, if both lateral and septal LA participate in the tachycardia, the tachycardia is likely to be peri-mitral reentry.22 Conversely, if both the posterior and anterior walls of the LA are within the circuit, the mechanism is likely to be ‘roof-dependent’ reentry. Downstream overdrive pacing is identified when pacing from electrodes that are activated ‘late’ results in orthodromic capture of the ‘earlier’ electrograms (Figure 4).23 This property is highly suggestive of a macro-reentrant mechanism. The post-pacing interval can also provide information about the distance of the pacing site from tachycardia location, regardless of the mechanism being focal, microreentrant or macro-reentrant.
High-density mapping for atypical flutters is valuable because it identifies complex patterns of activation, including dual-loop reentry and protected channels. Information from high-density mapping can be supplemented with judicious use of entrainment pacing, but some limitations of entrainment should be considered. Overdrive pacing can potentially interrupt or transform a tachycardia. Also, entrainment could potentially fail to identify a participating segment if rapid pacing causes further slowing of conduction in diseased myocardium, resulting in a long but misleading post-pacing interval.24
Once the tachycardia mechanism and dimensions have been defined, ablation should target a critical component of the tachycardia. Macro-reentrant ATs are ablated with linear lesions between unexcitable barriers including scar and anatomical structures. Ultra-high-density mapping now allows better resolution of scar, conduction block, zones of slow conduction and wave front collisions. As such, it may be possible to identify a ‘practical isthmus’, which is usually the narrowest part of the circuit and may be more effectively ablated than other components which require long linear lesions (Figure 5).25
Regardless of the location of macroreentry or the length of the ablative lesion, bidirectional conduction block is an important endpoint for ablation. Conduction block can be confirmed by the following criteria:26
- During pacing from one side of the line, activation mapping demonstrates a detour of the wave front around an anatomical barrier or scar, resulting in late activation of the opposite side.
- Differential pacing reveals that pacing further away from the line results in earlier activation of the opposite side.
- Double potentials may be demonstrated along the line, particularly when pacing from one side.
Right Atrial Macroreentry
Other than CTI-dependent atrial flutter, the most common form of macroreentry in the RA is free wall reentry (Figure 4). This circuit arises when scar is present in the RA free wall, usually as a result of right atriotomies but also as a spontaneous phenomenon in myopathic atria.27–30 Reentry in the RA free wall may coexist with another circuit, such as peri-tricuspid reentry or lower loop reentry around the inferior vena cava (IVC), to form a dual-loop tachycardia.31 A second form of macroreentry in the RA is known as upper loop reentry, which involves a circuit around the superior vena cava (SVC), possibly also encompassing patchy scar in the high RA adjacent to the SVC.
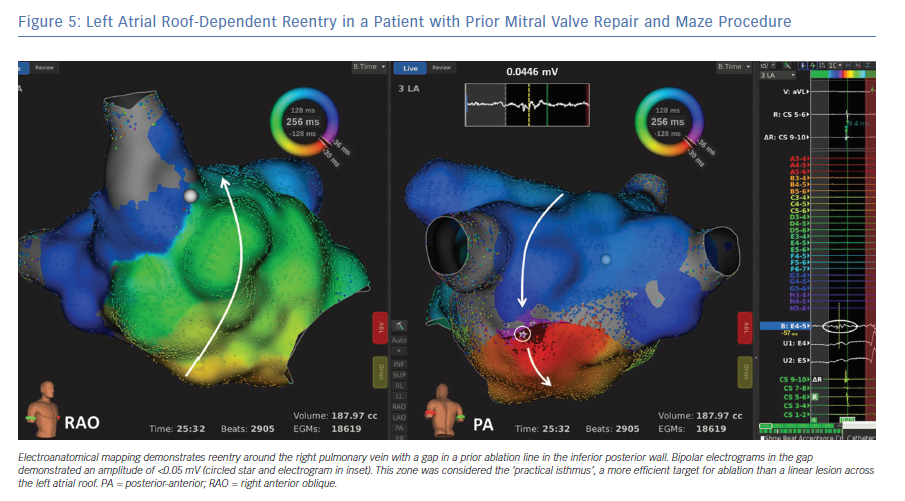
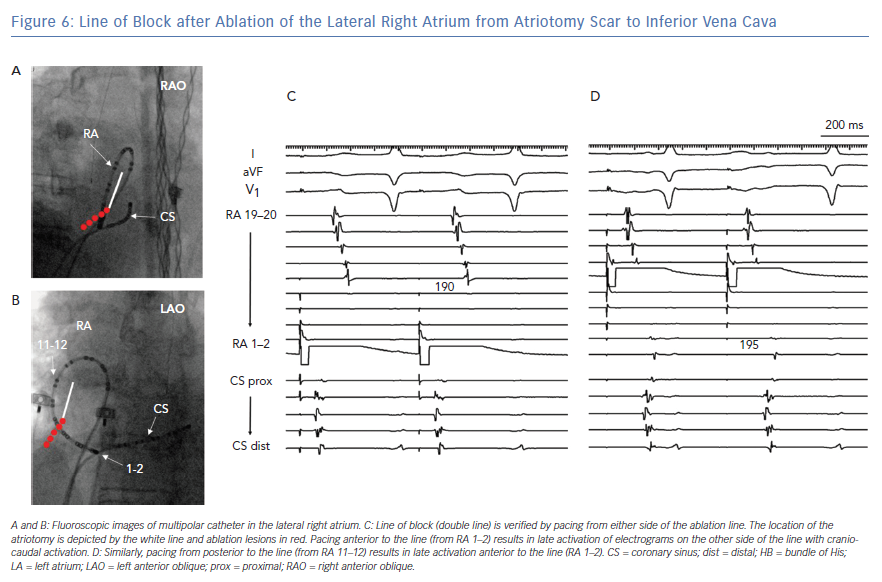
Ablation of lateral wall reentry in the RA is accomplished with a linear lesion between the preexisting scar and an anatomical boundary, such as the IVC, tricuspid annulus or SVC. When ablation is extended along the lateral wall to the IVC, conduction block can be verified with a multipolar catheter looped around the RA while pacing is performed both anterior and posterior to the line (Figure 6).32 Often, this lateral wall lesion is combined with ablation in the CTI. During ablation in the lateral RA, caution is required to avoid injury to the phrenic nerve.
Left Atrial Macroreentry
The vast majority of LA flutters occur in patients with atrial disease, such as those with prior atriotomies, previous catheter ablations or those who have developed LA myopathies. These arrhythmias take the form of single or dual loops, and multiple-loop reentry has also been described.
Reentry around the mitral annulus, known as peri-mitral reentry, is a common form of LA flutter. Recent studies using ultra-high-density mapping show that some peri-mitral circuits do not necessarily encompass the entire mitral annulus but detour around patches of scar in the LA.25 Peri-mitral reentry is usually interrupted by linear ablation in a ‘mitral isthmus’. The lateral mitral isthmus refers to the corridor between the mitral annulus and the left inferior pulmonary vein. Interruption of tachycardia in this location may require additional ablation in the coronary sinus to target epicardial fibers or connections between the coronary sinus and LA, including the ligament of Marshall.33 An alternative linear lesion may be created between the anterior mitral annulus and the left superior pulmonary vein or LA roof.34 Peri-mitral reentry can also be treated with linear ablation from the septal mitral annulus to the right superior pulmonary vein. It is worth noting that traditional criteria for block, with reversal of activation sequence and prolonged conduction times, might not distinguish slow conduction from complete block after ablation of peri-mitral reentry. In the lateral mitral isthmus, epicardial connections can result in slow conduction and the appearance of ‘pseudo-block’, but yet give rise to complex reentrant rhythms.35 These connections can be interrupted by targeting insertion sites in the proximal to mid coronary, or the ridge between the LA appendage and left pulmonary veins.
Another common form of LA flutter is reentry around an ipsilateral pair of pulmonary veins, also referred to as ‘roof-dependent LA flutter’ (Figure 5). Roof-dependent reentry is usually treated with linear ablation between the superior pulmonary veins. It is also possible to ablate between the inferior pulmonary veins across the posterior wall, but caution is required to avoid esophageal injury.
Many variants of macroreentry occur in the LA, depending on the particular pattern of native scar and prior ablation lesions. For example, reentrant circuits confined to the LA septum have been described,14 as have arrhythmias involving the coronary sinus or limited to the posterior wall.
Microreentrant Atrial Tachycardias
An interesting subset of ATs is best described as ‘micro-reentry’ or ‘localised reentry’. This mechanism occurs in patients with prior catheter ablation, prior atrial surgery and sometimes de novo in patients with atrial myopathy. Localised reentry is arbitrarily defined as a circuit with a diameter <2–3 cm.22 The circuits often occur adjacent to prior ablation lesions or near patchy areas of scar that are identified with electroanatomical mapping. These tachycardias characteristically demonstrate low-amplitude continuous electrograms encompassing >85% of the tachycardia cycle length (Figure 1D). Recent studies with ultra-high-density mapping show that localised atrial reentrant circuits may have multiple sequential zones of very slow conduction and occur in low-voltage areas of the atria.36 A notable feature of localised reentry is insensitivity to adenosine, a property which is common with macro-reentrant tachycardias (Figure 1C).37
Effective ablation of microreentrant ATs usually occurs at sites with highly fractionated, low-amplitude electrograms. These electrogram characteristics are highly sensitive but not specific for microreentrant circuits. In particular, low-amplitude and long-duration electrograms could be present at bystander sites which do not actively participate in the circuit. Also, electroanatomical maps can produce ‘pseudo’ localised reentry, which is actually related to incomplete rotation of an impulse and collision with an invading wave front.38 For this reason, entrainment is a useful manoeuvre to verify an active component of the circuit and appropriate target for ablation.
Outcomes After Catheter Ablation
Outcomes after ablation of ATs vary widely depending on the mechanism of arrhythmia, the degree of atrial myopathy and coexisting arrhythmias such as AF. The acute success rate for ablation of focal ATs is approximately 85–90%, and freedom from recurrence without antiarrhythmic drug therapy is 60–90%.39,40 Higher success is achievable in patients with single foci and structurally normal hearts.
For patients having ablation of post-incisional right ATs (many of whom also have CTI-dependent flutter), acute success of up to 96% has been reported with freedom from long-term tachyarrhythmia recurrences of 65%.29 Most of the recurrences are AF or ATs arising from different locations. In a series of patients having ablation of scar-related atypical atrial flutters in either atrium, acute success was approximately 90% and long-term success was 77%.41 Higher success was reported in those having prior catheter ablation or atrial surgery compared to those with idiopathic scar.
Conclusion
High-resolution mapping, in addition to entrainment responses, define the mechanisms of AT and identify sites of origin and critical isthmuses that are targets for ablation. With the aid of these mapping techniques, more focused lesions can be created to avoid ineffective ablations and proarrhythmia from excessive ablation. Understanding the atrial substrate for tachyarrhythmias may help minimise iatrogenic ATs that occur after catheter ablation of AF or following cardiac surgery.
Clinical Perspective
- Atrial tachycardias (ATs) can be classified as focal AT, macroreentry or localised reentry. The mechanisms may be distinguished by high-density mapping and responses to pharmacological agents such as adenosine.
- Focal ATs in patients with structurally normal hearts often arise from particular anatomical locations, such as the crista terminalis and valve annuli, including the para-Hisian region.
- High-density mapping of reentry may demonstrate an isthmus of slow conduction, which is a suitable target for ablation.
- Areas of slow conduction occur in patients with prior catheter ablation, cardiac surgery or atrial myopathy.
- These sites are characterised by electrograms of extremely low voltage, fractionation and long duration.